Context
Background
The Arctic Monitoring and Assessment Programme (AMAP) was established in 1991 to monitor identified pollution risks and their effects on the Arctic ecosystem (Fig1). Since 1997 a number of reports have been published with updates in 2002 and the latest in 20091-5. The AMAP is a working group of the Arctic Council. These AMAP working groups were established with the request to: (i) establish common strategies for AMAP monitoring and data handling; (ii) to produce integrated assessment reports on status, trends and state in the Arctic ecosystem; (iii) identify possible causes of changing modes; (iv) to measure the problems encountered, their possible causes and potential risk of the Arctic ecosystem instruments, including the Indigenous peoples and other Arctic inhabitants; and (v) to recommend actions necessary to reduce risks to the Arctic ecosystem.
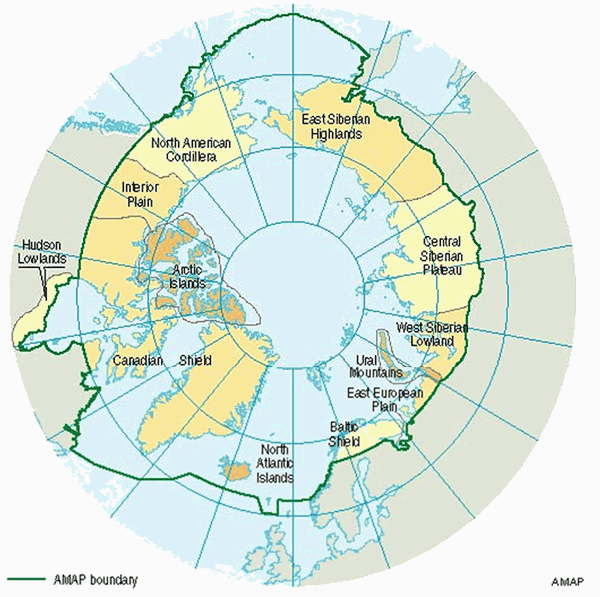
Figure 1: The Circumpolar area for Arctic Monitoring and Assessment Programme (AMAP) (www.amap.no).
The Arctic Council Ministers have given special priority to the potential effects of contaminants on the health of the Arctic populations, including the combined effects of environment contaminant mixtures (cocktail effects) that act with other potential environment stressors.
Since 1994 there has been biomonitoring of persistent contaminants and human health in the Arctic, and three scientific and international peer reviewed AMAP reports on human health were published in 1998, 2002 and 2009, respectively6-8. These reports have shown that the Arctic is closely linked with the rest of the world, that the Arctic regions are exposed to contaminants from sources far from the Arctic, and that the Arctic climate affects the global climate and vice versa.
The AMAP has assisted in the development, establishment, continuity and evaluation of protocols for heavy metals and persistent organic pollutants (POPs) for the United Nations Economic Commission for Europe (UN ECE) and the Stockholm Convention on POPs (Table 1)8.
Table 1: Chemicals currently listed under the Annexes to the Stockholm Convention and chemicals proposed to be added to the convention annexes8
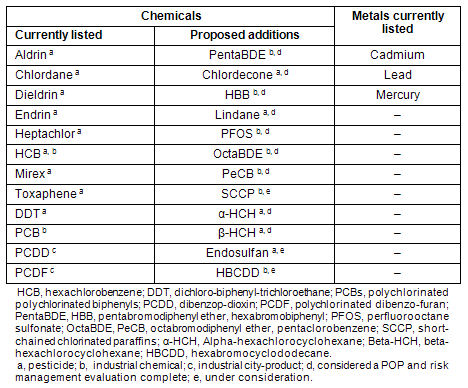
Levels of a number of POPs have decreased in the Arctic environment as a consequence of bans and restrictions on use and emissions within the Arctic and in other countries. POP contaminants that are primarily 'inherited' from previous use and emissions include polychlorinated biphenyls (PCBs), dichloro-biphenyl-trichloroethane (DDT), hexachlorobenzene (HCB), chlordane, dieldrin, toxaphene and, to some extent, dioxins.
These metabolically stable POPs are transported over long distances and found in the Arctic environment. They are bioaccumulated in animals as well as in humans, in particular in the adipose tissues because most POPs, such as PCBs, dioxins and organochlorine pesticides, are fat soluble. Transport to the Arctic is both through the atmosphere and via ocean currents, being slow in relation to atmospheric transport, which may delay the national response to environmental adjustments. Many new chemicals currently in commercial use have the same potential as 'old' known POPs to accumulate in the Arctic food chain; however, these are not yet subject to international agreements on the regulation. These chemicals include, for example, brominated flame retardants, fluorinated substances and polychlorinated naphthalenes, and the pesticide endosulfan. Other existing pesticides used and found in the Arctic include lindane (Gamma-HCH, hexachlorocyclohexane [HCH]), chlorpyrifos, chlorothalonil, dacthal, diazinon, diclofol, methoxychlor, and trifluralin8.
In the Arctic countries the Indigenous populations have a lower general health status than non-Indigenous populations, due to socio-economic conditions and their way of life; however, contaminants may play a contributing role in negative health effects among Indigenous peoples. A number of toxicological studies have shown that contaminants have the potential for causing adverse health effects in a population. Epidemiological studies in the Arctic have shown immunological, cardiovascular and reproductive effects due to contaminants in some Arctic populations. These results indicate that POPs, mercury and lead can affect health and that this is particularly so for children at lower levels than previously thought9. The genetic background to the Arctic population groups is different from Caucasian population groups, and these differences are likely to affect response to contaminants and sensitivity to an array of diseases10.
The traditional Greenlandic diet consists of a high intake of fish and marine animals, such as seal, whale and polar bear and seabirds. There is also a great dietary tradition of using organs and tissue such as livers, seal blubber and whale skin (mattaq), which are all in some way nutritious. However, there is a dilemma in such practices for animal fats may also contain high concentrations of POPs7,8. A general observation has been made to clearly link age and intake of traditional diet with blood POP levels, with lifestyle factors such as smoking further increasing the bioaccumulation of POPs8.
During recent years there has been a shift away from the intake of traditional Greenlandic diet to imported food from supermarkets. This is particularly pronounced in major towns such as Nuuk and Sisimiut on the west coast and women seem to have a higher intake of imported food. However, the traditional diet still plays a major social, cultural, economic and dietary role of the Indigenous population7,8. The observed shift from traditional to imported diet is reflected in findings that fewer women of childbearing age are exceeding international recommendations for blood level of PCBs, mercury and lead8.
A number of studies have published information on the mechanisms whereby environmental chemicals affect metabolism. As in other parts of the world obesity is increasing in Arctic areas, and thus also the risk of cardiovascular diseases and diabetes11. Toxicological population studies in the Arctic have indicated the effects of contaminants on reproduction, pregnancy rates and fetal development9. An overview of the toxicological properties of POPs and related health effects for Arctic populations was published in 200312. Environmental and human biomonitoring programmes have been conducted in the Arctic1-8( Fig2). Since 2000, parallel studies have been carried out in Greenland on the human monitoring of biomarkers for POP exposure and biomarkers for POP effects, focusing on hormone disruptive potentials and some genetic sensitivity biomarkers. This review will give an overview of these Greenlandic studies during the period 2000 to 2006.
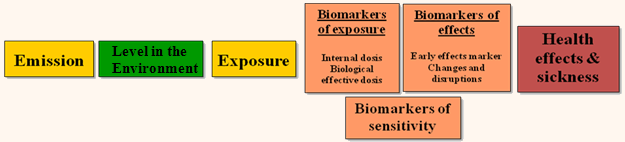
Figure 2: Determination of bio-monitoring factors can integrate environment exposure, effects, individual sensitivity and health risks.
Methods
Study population: As described13,14 participants in the studies were randomly selected from the national register. All participants were descendants of Inuit, defined as having more than two grandparents born in Greenland. The participation rates were generally close to 90-100%. The study was designed to include 50 men and 50 women aged between 18 and 50 years from each district. In some cases, blood sampling and handling resulted in fewer samples from some districts for the biomarker of effect analyses. All participants completed a standard interview on demographic and lifestyle parameters. Blood samples were taken for the determination of POPs, fatty acids and xenobiotic hormone-disrupting potential of POPs, and stored at -800C until analysis.
POPs and fatty acids: Plasma samples were analyzed for the following POPs: the cis-, trans-and oxy-chlordane, p,p'- DDE, p,p'-DDT, hexachlorobenzene (HCB), β -hexachlorocyclohexane (β -HCH), mirex, toxaphene 26, toxaphene 50 and 14 PCB congeners (CB28, CB52, CB99, CB101, CB105, CB118, CB128, CB138, CB153, CB156, CB170, CB180, CB183, CB187) at the certified laboratory, Le Centre de Toxichologie, Sainte Foy, Quebec, Canada as described15. All specific POPs were adjusted to the sample plasma lipid concentration and given as μg/kg lipid. Fatty acid profiles were determined as plasma phosphorlipids by the Biology Department, University of Guelph, Canada. The n-3 polyunsaturated fatty acids was listed as sum of C18: 3, n-3, 8 to 20: 4, n-3, 8 to 20: 5, n-3, C22: 5, n-3 and C22: 6, n-3, and n-6 fatty acids as sum of C18: 2, n-6, C18: 3, n-6, 8 to 20: 2, n-6, 8 to 20: 3, n-6 and 8 to 20: 4, n-6.
SPE-HPLC extraction and fractionation of serum samples: In order to isolate the serum fraction that contains the bioaccumulated fat soluble POPs a solid-phase-extraction (SPE); followed by high performance liquid chromatography (HPLC) fractionation of 3.6 mL serum was performed as described16. The first HPLC fraction (F1: 0.00- 5.30 min.) was defined to include most POPs separated and free from natural endogenous hormones. This F1 SPE-HPLC fraction was vaporized and stored at -800C until analysis for POP related xenoestrogene and xenoandrogene effects via estrogen receptor (ER) and androgen receptor (AR) reporter gene transactivation. In parallel, at least once a week control serum samples from men and women from the blood bank at Aarhus hospital, DK were analyzed. The procedures for thawing and analysis of the SPE-HPLC extracts are described17-18.
Hormone receptor transactivation of the luciferase reporter gene expression: The SPE-HPLC F1 serum extracts were analyzed for their potential to affect ER and AR transactivation in MVLN and Chinese Hamster Ovary (CHO-k1) cell lines, respectively, as described17-18. Serum F1 extracts was analyzed by cells exposure alone reflecting the agonistic power (specified by XER and XAR, respectively). In addition, as a physiological mimic, the F1 extracts by co-exposure with the respective high potent receptor ligand (17β -estradiol [E2]) for ER and methyltrienolone (R1881) for AR (specified as XERcomp and XARcomp, respectively) reflecting the potential of the F1 serum to compete / antagonize the potent receptor ligand induced activity as well as the ability to further increase the ligand induced receptor activity. The receptor transactivated luciferase activity is given as relative luciferase units per micrograms cell protein (RLU/μg protein) corresponding to a response of 0.31 mL serum.
Dioxin-like serum activity: Extraction of serum POPs for determination of dioxin-like activity was performed at the certified laboratory Le Centre de Toxichologie, Sainte Foy, Quebec, Canada as described19,20. Serum dioxin-like activity was determined using the aryl hydrocarbon receptor (AhR) transactivation of the luciferase reporter gene expression in the stable transfected mouse hepatoma cell line Hepa1.12cR as described19,20. The serum extract induced agonistic AhR-luciferase activity is expressed as AhR-TCDD equivalents (AhR-TEQ) (TCDD; 2, 3, 7, 8-tetrachlorodibenzo-p-dioxin); for the calculation see19,20.
Statistical analyses: Statistical analyses were conducted using SPSS 13.0 (SPSS Inc, Chicago, IL, USA). The term statistical significance level is used to denote by a p-value ≤0.05, as described17-20.
Issue and Lessons learned
Biomarkers for POP and metal exposures
Figure 3 shows Greenland with geographical located columns showing the relative total sum of POPs exposure biomarkers, and within the column the relative profile of each POP group determined in human serum typically including the 50 men and 50 women from each district14. It is evident that both the sum of PCBs and the sum of organochlorine pesticides are highest in the East Greenlandic district (EG) (Scoresbysund) followed by Tasiilaq (EG) and the Thule district in Northern Greenland (NG) and Narssaq in South Greenland (SG). For Qeqertarsuaq (Godhavn, Disco Bay) and Nuuk (West Greenland, WG) the sum of PCBs and organochlorine pesticides are very similar. However, it should be noted that the data for Nuuk men are atypical as they were older and had a higher dietary intake of seabirds and, therefore, a higher level of serum POP concentration. In general the POP levels in Nuuk and Sisimiut, the two most Westernized cities in terms of diet, are at the same level and lower than for POP measurements in the other Greenlandic districts. What also should be noted is that selenium and mercury serum concentrations were found at the highest level in the Thule area. With respect to serum lead, the highest levels were found in Scoresbysund.
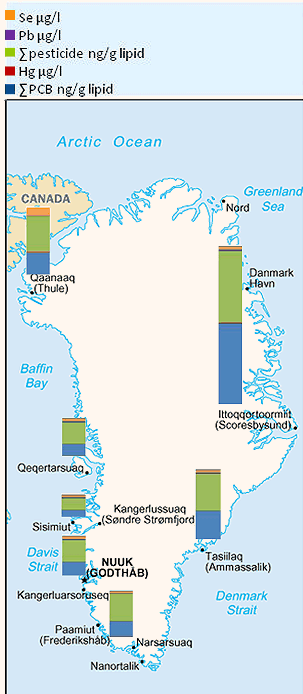
Figure 3: Human POP biomarkers for exposure in Greenland. The columns illustrate the relative ∑ POPs and the different POP profiles and metal concentrations within the columns in the different Greenlandic districts for the period 2000-2006. Note that the concentration units are different because ∑ PCBs and ∑ pesticides (details in Table 2) are given in ng/g lipid and metals in µg/l serum.
Table 2: Human POP biomarkers for exposure in Greenland data for Figure 3
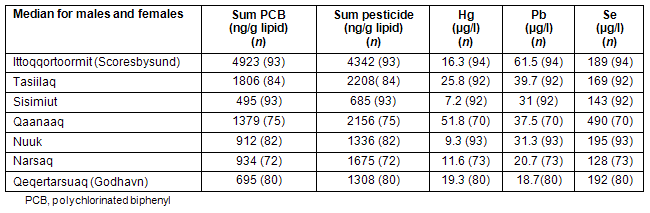
Biomarkers for receptor effects
It is well known today that the level and profiles for the various POP groups vary among Greenland districts. Similarly, a number of toxicological studies have shown that individual POPs have very different biological effects and potential. For example some PCB congeners have estrogen potential (eg some hydroxy-PCBs) while others are antiestrogene (eg PCB153, PCB180, PCB138) and antiandrogene (PCB-138)21; some have dioxin-like potentials (eg PCB126) and, likewise, for organochlorine pesticides there has been reported both estrogenic potentials (eg toxaphene, β-HCH, DDT and DDE) and antiandrogene effects (eg DDE)9,12,21-23. As a result of these different and often opposed directed biological effects and potentials of POPs, it is very difficult, if not impossible, to predict a given biological effect of the very complex mixtures (cocktails) of POPs that actually exists in the human body (blood).
With the aim to investigating the toxicological and endocrine disrupting potential of the actual mixture of POPs in the blood of Greenlandic Inuit we have, as a biomarker for effect, determined the integrated impact of serum POP extracts that affects the function of hormone receptors ER and AR, as well as AhR mediated dioxin-like activity in serum. Presently, the sum POP-related effects on receptor activities are determined for Nuuk, Sisimiut, Tasiilaq and Qaanaaq24,25; whereas the effect biomarkers for Scoresbysund (EG), Godhavn (WG) and Narssaq (SG) are in progress.
Figure 4 shows the serum POP related receptor activities for Nuuk (WG), Sisimiut (WG), Tasiilaq (EG) and Qaanaaq (NG). For ER activity the serum POPs elicited in general an antagonistic (21-81% of the samples) effect, where the activity inhibition was in the order: Tasiilaq > Sisimiut > Qaanaaq > Nuuk. Few of the serum POP extracts elicited agonistic ER activity (< 6%, Qaanaaq > 14%). There were observed differences both among districts and between sexes, which in turn was district dependent24.
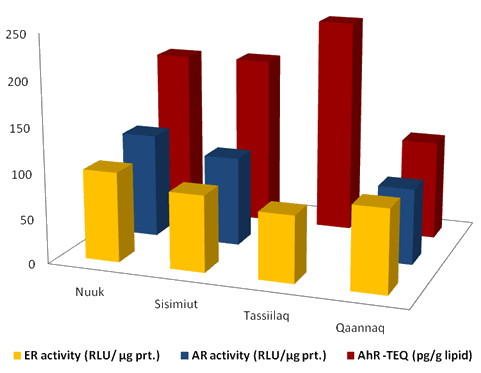
Figure 4: Diagram for serum POP related receptor activities in Greenlandic Inuit. ER, estrogen receptor; AR, androgen receptor; AhR, aryl hydrocarbon receptor; RLU/ug prt; relative light units per microgram cell protein.
Table 3: Serum POP related receptor activities in Greenlandic Inuit data for Figure 4.
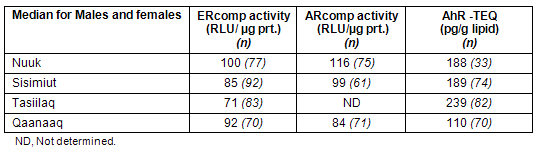
Preliminary data for serum POP related ER activity for Scoresbysund, Godhavn and Narssaq shows a trend of higher frequency of serum samples from East Greenland (Scorebysund) eliciting antiestrogen activity compared with West Greenland (Godhavn and Narssaq) (EC Bonefeld-Jørgensen; unpubl.data, 2010). The trend was similar for men and women. These data supports observations on the antiestrogene potentials of POPs as the highest ∑ POP concentrations were found in Scorebysund and Tasiilaq (Fig3).
Also for the AR activity a district dependent difference in serum POP effect on the AR activity was observed with the following order of medians: Nuuk > Sisimiut > Qaanaaq (Fig4). In general the AR activity was higher for men than women. For men from Nuuk and Sisimiut a significant induced AR agonistic activity was observed, which was in accordance with the frequency of samples that could further increase the AR ligand (R1881) induced receptor activity24. In contrast, a higher frequency of serum extracts that inhibited/antagonized the induced AR activity were seen for women in Nuuk, Sisimiut, and in Qaanaaq for both men and women24.
The POP related dioxin-like serum activities is given by TCDD toxicological equivalence (AhR-TEQ). The following order of AhR-TEQ medians was observed: Tasiilaq ≥ Sisimiut ≥ Nuuk > Qaanaaq (Fig4). The frequency of serum extracts representing agonistics AhR activity was 71-100% and similar levels were found in women and men25.
By comparison of the effects of the serum POP mixtures on the three receptor activities (Fig4) there is a trend towards a relationship between the higher AhR-TEQ (dioxin activity) level the lower ER activity which supports the reported antiestrogene activity of dioxins.
The European Union supported project Inuendo which aimed to examine whether there is a correlation between human fertility and exposure to POPs, using the POP exposure biomarkers PCB-153 and p,p'-DDE. The project involved population groups of fertile men from Europe (Kharkive, Ukraine; Warsaw, Poland; fishermen of the Swedish East Coast; and various Greenlandic districts)26. A significant higher serum concentration of the two POP exposure markers was found for Inuit (Fig5). Concerning serum POP related receptor effect biomarkers, we found a significant lower ER and AhR activity level and a trend towards higher AR activity for Greenlandic serum samples in relation to the European samples17-19. Unexpectedly, a significant lower level of DNA damage was found in sperm DNA from Inuit compared with European samples27. Further studies are required to identify possible factors involved in the correlations found between POP related receptor effects and sperm DNA damage28-29.
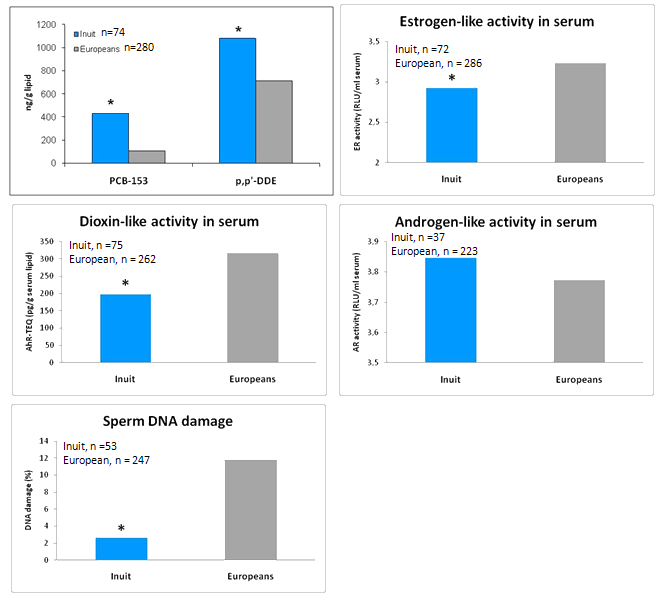
Figure 5: Biomarkers for exposure and biomarkers for effects in Inuit and European males. The POP proxy markers (PCB-153 and p, p'-DDE) are given as nanogram per gram lipid. Dioxin-like activity is given as aryl hydrocarbon receptor - TCDD toxicological equivalents (AhR-TEQ). ER, AR activities are given as relative light unit per milliliter serum. P <0.05, Inuit vs Europeans.
Biomarkers for sensitivity
The genetic background can be decisive for an individual's sensitivity for exposure to environment contaminants. Genetic studies of mitochondrial DNA have supported the perception of Inuit East Asian kinship10, and we have unpublished genetic data (2010) on Greenlandic Inuit that supports this kinship. Few genetic studies are reported on gene polymorphisms in the Indigenous Arctic population (Inuit) in particular in relation to the concepts of disease risk. Studies from 1950 to 1974 showed that of 11 examples, only apoplexy and epilepsy were more frequent in Inuit, whereas gastric ulcer, acute heart attack, psoriasis, asthma, diabetes, bronchial thyroid toxicoses and multiple sclerosis were lower than expected compared with the Danish population10.
Approximately 80% of all cancers are, based on epidemiologic studies, suspected to be related to environmental exposures. Cancer sensitivity may be a result of differences in the genetic background of metabolism, DNA repair and changes in gene expression of tumor related genes10. Therefore, gene polymorphisms in, for example, metabolizing enzymes such as the P450 are suspected to influence sensitivity to environmental carcinogens. There are studies which have indicated coherence between gene polymorphisms in P4501A genes, the level of POPs and the risk for development of breast cancer in Caucasians10.
Epigenetic changes are a new paradigm in toxicology. It is a phenomenon that allows inheritable transfer of acquired DNA modifications without any changes to the primary DNA sequence. It is reflected by changes in the control of gene activity which originate from the interplay between DNA methylation, histone modification and RNA mediated pathways. Epigenetic regulation is a part of the normal development and differentiation, but by disruption it may cause diseases such as cancer10. Exposure to, for example, persistent environmental contaminants such as PCBs and polybrominated biphenyls (PBBs) can play a role in two-stage cancer models involving DNA methylation. A small Greenlandic study that included the different districts showed a positive correlation between POP serum concentrations and global hypomethylation of DNA30, suspected to be involved in cancer risks. Further studies are required in order to elucidate these cellular and biological effects in relation to health.
Summary, conclusion and perspectives
Biomonitoring studies for exposure biomarkers in Greenland have shown that there are geographical differences in the bioaccumulated POP levels in the blood of Inuit from different districts. These differences are primarily attributable to diet and lifestyle, higher levels of traditional Greenlandic diet (eg seal, whale, polar bear, seabirds) and smoking and are reflected by a higher concentration of POPs in the blood. The highest POP values were found on the east coast of Greenland. Biomonitoring studies for receptor effect showed a general correlation between high serum POP concentration and inhibited ER and AhR activity; however, for men in the two West Greenlandic cities Nuuk and Sisimiut a trend towards increased AR activity was observed. In contrast, an inverse trend between dioxin-like AhR activity and ER activity supports the perception of that dioxins exert an antiestrogen effect. So, in conclusion, the actual cocktail of serum POPs in the Greenlandic Inuit has endocrine disrupting potential.
Biomarkers for POP exposure showed that men from Greenland (Inuit) had significant higher serum POP levels compared with European men from Sweden, Ukraine and Poland. Unexpectedly, in the same study groups it was observed that Inuit had a lower level of DNA damage in sperm in relation to the Europeans. Further studies are needed to elucidate whether there is a relationship between the impact of serum POPs on the activity of hormone and/or dioxin receptors and the level of sperm DNA damage. However, it is known that selenium and n-3 unsaturated fatty acids are important factors in production of semen.
The problem of traditional Greenlandic diet versus imported diet is often referred as the 'Arctic dilemma'. The dilemma is that with the intake of the Greenlandic traditional marine diet, including a number of important trace elements/antioxidants such as selenium and marine unsaturated fatty acids shown to have favorable effects on health, bioaccumulated POPs are also consumed, which is suspected to have a number of adverse health effects. Nevertheless, there are a number of studies which suggest that an intake of a more Western diet can lead to other health risks, such as increase in the prevalence of the metabolic syndrome and its sequels. However, these contexts are still to be confirmed and require continued studies involving determinations of biomarkers for exposure and effects, epigenetic factors, the determination of relevant genetic polymorphisms, and case-control contexts. Transgenerational studies (mother-child cohorts) are of high importance in order to elucidate the effect of exposure to environmental contaminants on fetal development and health risks. It should finally be mentioned that there is a need for the development and use of new biomarkers for effects such as for the potential of POPs to inhibit the immune system, as well as biomarkers for the development of central nervous system.
Acknowledgements
The authors thank all those who have contributed to the reported data. These include researchers associated with the Unit for Cellular & Molecular Toxicology, Center for Arctic Environment Medicine, School for Public Health: Assistant Professor Manhai Long, postdoctoral researchers Tanja Krüger and Mandana Ghisari, PhD student Philip S Hjelmborg, research assistant Yi Cao, and laboratory assistant Birgitte Sloth Andersen. Also thanked are Adjunct Associate Professor Henning Sloth Pedersen, Associate Professor Bente Deutch, Associate Professor Jens C Hansen and the colleagues in the Inuendo project www.inuendo.dk. The studies were carried out with financial support from the Danish Ministry of the Environment (DANCEA), the Health Science Research Council, Aarhus University Research Fund, The national Institute of Health (NIH, United States), and the European Union.
References
1. Arctic Monitoring and Assessment Programme. Arctic pollution issues: a state of the arctic environment report, xii,188. (Online) 1997. Available: www.amap.no (Accessed 12 March 2009).
2. Arctic Monitoring and Assessment Programme. Arctic pollution 2002, xii,112. (Online) 2002. Available: www.amap.no (Accessed 12 March 2009).
3. ACIA. Impacts of a warming arctic. Arctic climate impact assessment. Cambridge University Press, 2004; 139.
4. Arctic Monitoring and Assessment Programme. Arctic pollution 2006: acidification and Arctic haze, xii,112. (Online) 2006. Available: www.amap.no (Accessed 12 March 2009).
5. Arctic Monitoring and Assessment Programme. Arctic oil and gas 2007, xiii,40. (Online) 2008. Available: www.amap.no (Accessed 12 March 2009).
6. Arctic Monitoring and Assessment Programme. AMAP assessment report: Arctic pollution issues, xii,859. (Online) 1998. Available: www.amap.no (Accessed 12 March 2009).
7. Arctic Monitoring and Assessment Programme. AMAP assessment 2002: human health in the Arctic, xiii,137. (Online) 2003. Available: www.amap.no (Accessed 12 March 2009).
8. Arctic Monitoring and Assessment Programme. Arctic Pollution 2009, xi,83. (Online) 2009. Available: www.amap.no (Accessed 12 March 2009).
9. Gilman A, Ayotte P, Dewailly E, Dudarev A, Bonefeld-Jørgensen EC, Muckle G et al. Public health and the effects of contaminants. In: The Arctic Monitoring and Assessment Report :Human health in the Arctic, xiv,256; chapter 8. (Online) 2009. Available: www.amap.no (Accessed 12 March 2009).
10. Bonefeld-Jørgensen EC. Genetic and contaminants. In: The Arctic Monitoring and Assessment Report: Human health in the Arctic, xiv,256; chapter 6. (Online) 2009. Available: www.amap.no (Accessed 12 March 2009).
11. Arctic Monitoring and Assessment Programme. Interactions between contaminants and nutrients, xiv,256; chapter 7. (Online) 2009. Available: www.amap.no (Accessed 12 March 2009).
12. Bonefeld-Jørgensen EC, Ayotte P. Toxicological properties of POPs and related health effects of concern for the arctic populations In: Human health in the Arctic, xiv,137; chapter 6. (Online) 2002. Available: www.amap.no (Accessed 12 March 2009).
13. Deutch B, Dyerberg J, Pedersen HS, Asmund G, Moller P, Hansen JC. Dietary composition and contaminants in north Greenland, in the 1970s and 2004. Science of Total Environment 2006; (2-3): 372-381.
14. Deutch B, Pedersen HS, Asmund G, Hansen JC. Contaminants, diet, plasma fatty acids and smoking in Greenland 1999-2005. Science of Total Environment 2007; 372(2-3): 486-496.
15. Butler Walker J, Seddon L, McMullen E, Houseman J, Tofflemire K, Corriveau A et al. Organo chlorine levels in maternal and umbilical cord blood plasma in Arctic Canada. Science of Total Environment 2003; 302(1-3): 27-52.
16. Hjelmborg PS, Ghisari M, Bonefeld-Jorgensen EC. SPE-HPLC purification of endocrine-disrupting compounds from human serum for assessment of xenoestrogenic activity. Analytical and Bioanalytical Chemistry 2006; 385(5): 875-887.
17. Bonefeld-Jorgensen EC, Hjelmborg PS, Reinert TS, Andersen BS, Lesovoy V, Lindh CH et al. Xenoestrogenic activity in blood of European and Inuit populations. Environmental Health 2006; 5(1): 12.
18. Krüger T, Hjelmborg PS, Jönsson BAG, Hagmar L, Giwercman A, Manicardi GC et al. Xeno-androgenic activity in serum differs across European and Inuit populations. Environmental Health Perspectives 2007; 115(Suppl1): 21-27.
19. Long M, Andersen BS, Lindh CH, Hagmar L, Giwercman A, Manicardi GC. Dioxin-like activities in blood across European and Inuit populations. Environmental Health 2006; 25(5): 14.
20. Long M, Deutch B, Bonefeld-Jorgensen EC. AhR transcriptional activity in serum of Inuits across Greenlandic districts. Environmental Health 2007; 23(6): 32.
21. Bonefeld-Jørgensen, EC, Andersen HR, Rasmussen TH, Vinggaard AM. Effects of highly biomagnified polychlorinated biphenyl congeners on androgen and estrogen receptor activity. Toxicology 2001; 158(3): 141-153.
22. Jørgensen ECB, Autrup H, Hansen JC. Effect of toxaphene on estrogen receptor functions in human breast cancer cells. Carcinogenesis 1997; 18: 1651-1654.
23. Rasmussen TH, Nielsen F, Andersen HR, Nielsen JB, Weihe P, Grandjean P. Assessment of xenoestrogenic exposure by a biomarker approach: application of the E-Screen bioassay to determine estrogenic response of serum extracts. Environmental Health 2003; 15(1): 12.
24. Krüger T, Ghisari M, Hjelmborg PS, Deutch B, Bonefeld-Jorgensen EC. Xenohormone transactivities are inversely associated to serum POPs in Inuit. Environmental Health 2008; 15: 7-38.
25. Long M, Deutch B, Bonefeld-Jorgensen EC. AhR transcriptional activity in serum of Inuits across Greenlandic districts. Environmental Health 2007; 23(1): 32.
26. Bonde JP, Toft G, Rylander L, Rignell-Hydbom A, Giwercman A, Spano M et al. Fertility and markers of male reproductive function in Inuit and European populations spanning large contrasts in blood levels of persistent organochlorines. Environmental Health Perspective. 2008; 116(3): 269-77. (Review). Erratum in: Environmental Health Perspectives 2008; 116(3): 276.
27. Stronati A, Manicardi GC, Cecati M, Bordicchia M, Ferrante L, Spano M et al. Relationships between sperm DNA fragmentation, sperm apoptotic markers and serum levels of CB-153 and p,p'-DDE in European and Inuit populations. Reproduction 2006; 132(6): 949-958.
28. Long M, Stronati A, Bizzaro D, Krüger T, Manicardi G-C, Hjelmborg PS et al. Relation between serum xenobiotic induced receptor activities and DNA damage and sperm apoptotic markers in European and Inuit populations. Reproduction 2007; 133(2): 517-30.
29. Krüger T, Spanò M, Long M, Eleuteri P, Rescia M, Hjelmborg PS et al. Xenobiotic activity in blood and sperm chromatin integrity in European and Inuit populations. Molecular Reproduction and Development 2008; 75(4): 669-680.
30. Rusiecki JA, Baccarelli A, Bollati V, Tarantini L, Moore L, Bonefeld-Jorgensen EC. Global DNA hypomethylation is associated with high serum persistent organic pollutants in Greenlandic Inuit. Environmental Health Perspectives 2008; 116(11): 1547-1552.